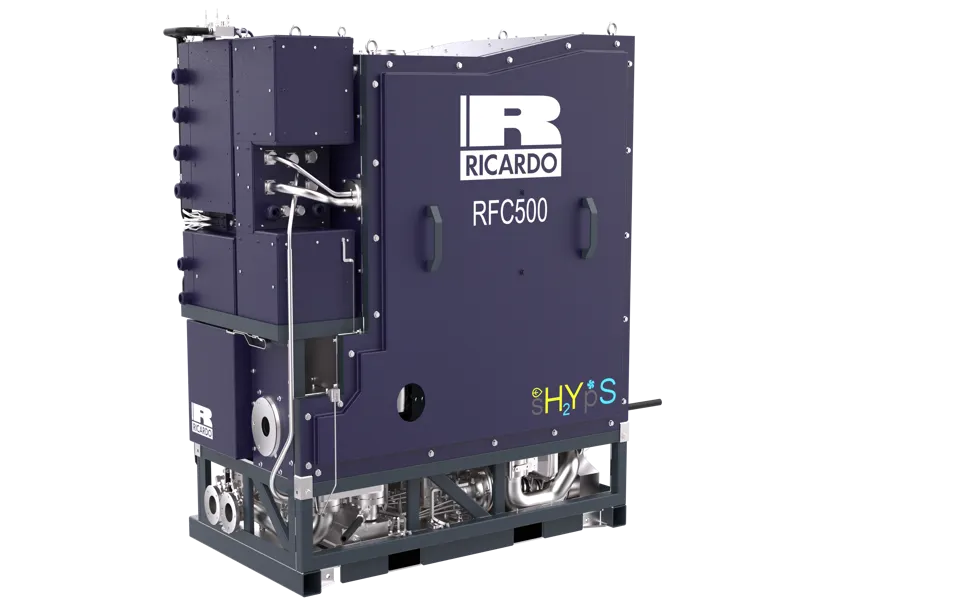
Shahin Hessami is a global fuel cell technical expert at Ricardo. He comments on how Ricardo is developing high power, high power-density, multi-stack fuel cell solutions for a variety of hydrogen applications.
Multi-stack fuel cell systems are developed through the integration of multiple fuel cell stacks with a single, optimised, Balance of Plant (BoP). There are key advantages of multi-stack hydrogen fuel cell systems, they can offer significantly increased power density, improved system level efficiency and the potential to reduce system cost by reducing part count. Such solutions can offer real benefits to a variety of applications including those in the marine, off-highway, commercial vehicle, and aerospace industries.
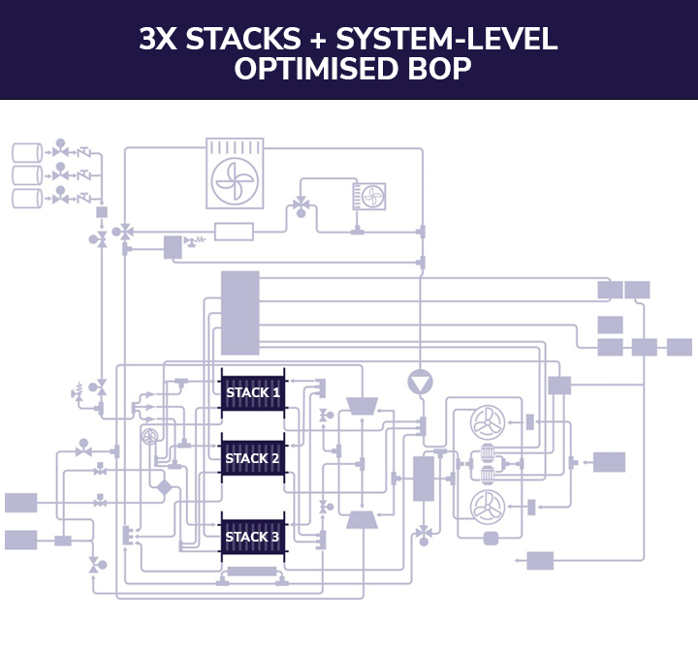
Ricardo is a key innovator within multi-stack fuel cell system applications, delivering bespoke integrated solutions to customers. Ricardo’s full, turnkey capabilities including simulation, build and full system testing combined with our experience in the design, control and integration of fuel cell systems, means that we are solving even the most complex of challenges.
Through innovative design, integration and packaging, Ricardo can not only address the challenges posed by space and weight constraints but also position multi-stack technology as a high-performing, compact, and versatile solution for applications that demand substantial power levels.
In conventional fuel cell systems, each individual stack typically requires a dedicated set of components, or BoP, including air compressors, hydrogen recirculating blowers and cooling pumps. Power to these systems is limited by the maximum size of a single fuel cell stack, typically in the range of 70 to 100kW net. However, for applications that require 300kW, and more, a multi-stack fuel cell system can be a good solution. Due to the size of these applications, a bespoke BoP can be very beneficial, providing an engineering solution that combines various supporting and auxiliary components of the power plant system to produce power. The right system level solution keeps everything running stably and efficiently, ensuring the required performance is achieved. A multi-stack solution does not enhance the performance of the individual hydrogen fuel cells; however, it does reduce system level parasitic losses.
Crucial to the BoP design is that despite a reduction in mass and size, it provides the correct level of reactants and coolant to all of the fuel cell stacks whilst maintaining or increasing overall efficiency. The integration of a single BoP also contributes to the overall simplification of the system architecture, with a positive impact on maintenance, cost effectiveness and ease of deployment. This simplification incorporates a reduction in fluid connections (i.e. tubing, hoses, fitting and flanges), filters, HV cables and connectors, low voltage harnesses and data/signal lines and motors and drivers for the BoP components.
There are manageable risks associated with the integration of multi-stack hydrogen fuel cell systems, notably, the uniform distribution of reactants and coolant between the fuel cell stacks. At Ricardo we use our experience and engineering expertise to design flow distribution systems to help mitigate those risks. However, several additional challenges are inherent in the integration of multi-stack fuel cell system, including management of failures, and the ability to maintain power output if needed. These challenges underscore the importance of robust backup and fail-safe systems to ensure continuous operation should a component fail.
Other risks include complex power electronics and controls, supplier hardware availability and maturity. All of which pose a potential challenge if not managed correctly as part of the design and integration process. The complexities in power electronics and controls, means that optimal performance is reliant on ensuring seamless interplay between components, through sophisticated systems. To arrive at a multi-stack fuel system that delivers the required performance, whether that be in terms of efficiency, durability, peak power, or transient response, necessitates consideration of appropriate control architecture, which must be incorporated at the concept stage. For example, to understand where independent control is necessary or beneficial, or conversely where it may be detrimental in terms of overall system complexity.
For some applications, benefits identified through simulation may justify the use of higher quality sensors. Alternatively for lower-cost applications, simulation studies may give confidence in the deletion of sensors/actuators and the overall simplification of the BoP. Throughout the development process good multi-domain models of the fuel cell system, including the controller, are crucial. These models are important at the concept phase to validate architectural decisions, and later in the development of intelligent offline calibration.
Since higher fidelity models are increasingly useful, a good methodology for capturing data from the various levels of test, to improve model quality, is important. The capability to rapidly deploy prototype control systems and targeted data acquisition can also improve test efficiency.
A comprehensive electronic strategy will ensure successful integration of these components within the multi-stack fuel cell solution, while also helping to maximise performance, reliability, and efficiency.
Advanced analysis and testing methods for multi-stack hydrogen fuel cells also paves the way for broader adoptions of the technology. More recently, this has included:
-
The adoption of advanced two-phase CFD to analyse distribution of water vapour and condensation in stack manifolds. This precision in understanding fluid dynamics contributes to optimising the design of multi-stack configurations and ensuring efficient water management in the system.
-
Use of statistical analysis of end of the line stack performance in selecting optimal stack groups. This data driven approach aids in identifying and utilising stacks with superior performance characteristics, contributing to overall system efficiency and reliability.
-
Real time analysis of stack cell voltage deviations to identify performance trend during prototype testing. This dynamic analysis helps fine-tune the control strategies and enhance the overall performance of multi-stack hydrogen fuel cells.
-
Fault emulating test strategies, in simulation and on the test bed, to test durability of flow balancing concepts and sensitivity to noise factors. This proactive approach ensures robustness and reliability in real-world scenarios, contributing to the technology's readiness for diverse applications.
Looking towards a future where a broader adoption of multi-stack fuel cell technology exists, Ricardo’s role is to enable the scale-up of this technology and its introduction into new applications. The developments listed, including advanced analysis and testing methods, serve as essential tools to enhance our engineering solutions. They contribute to the reliability and scalability of fuel cell systems, making them more accessible for a wider range of applications.
The focus for developing multi-stack fuel cell technology will lean towards enhancing efficiency and affordability. This is crucial to unlocking the full potential of hydrogen fuel cells across a wider range of applications. Some of the key considerations will include:
-
BoP component supply base: the development of a robust supply base for BoP components is essential. For instance, multi-stack fuel cell systems require H2 and air valves that can deliver four times more flow compared to the current valves used on a 100kW unit fuel cell system. Improvements in the supply chain for components will contribute to the overall enhancement of multi-stack systems.
-
Power electronics, control valves and control techniques for load balancing between fuel cell stacks and measurement and control of H2 flow to the stacks. Further research in this area will focus on developing sophisticated control strategies to manage power distribution effectively, ensuring each stack operates at its optimal condition.
-
Reliable hydrogen sensors that can operate across a wide range of concentrations and in wet environments. Advances in sensor technology will enhance the accuracy and responsiveness of monitoring hydrogen levels, contributing to the safety and performance of fuel cell systems.
-
Future research is also likely to explore innovative sensors and techniques to measure and regulate hydration levels effectively, which is essential for maintaining optimal conditions within the stacks, to improve efficiency and durability.
The continuous development of technology will support the future potential of multi-stack fuel cells in a variety of applications, creating a sustainable and energy efficient solution for future mobility.